U.S. and international space authorities have ambitious manned spaceflight schedules through the mid-21st century, which will call for enabling technologies ranging from radiation-hardened microelectronics components, to advanced robotics, and to habitat enhancements like water recycling, heating and cooling, and long-term food production and storage.
Perhaps the most important life- and mission-critical technologies will involve radiation-hardened and radiation-tolerant electronics, which will help ensure that crucial subsystems like communications, navigation, orbital-control, and life-support systems can survive and operate through the kinds of radiation levels found in high-Earth orbits, and into Earth's solar system.
"The biggest differentiator for manned vs. unmanned space flight is radiation hardened electronics for single-effects performance," explains Leonard Leslie, vice president of engineering at power electronics specialist VPT Inc. in Blacksburg, Va.
Radiation-hardened electronics
Single-event effects are not immediately the most destructive radiation things that can happen to electronics in space, but can cause quick malfunctions in computer memory and processing that can be difficult to detect and diagnose. The most damaging radiation effects are latchup and burnout, which can cause catastrophic electronics failures.
Single-event effects, on the other hand, are more sneaky. A single-effect event happens when a charged radiation particle hits an electronic device like a memory, processor, or power controller just right and causes a bit flip -- essentially changing a piece of digital information from a one to a zero -- and produce unreliable information.
"Single-events occur from a particle, and the response can occur the moment the spacecraft is out of the atmosphere," says VPT's Leslie. "There is a wide range of single events, from small transients or corruption of computer memory or instruction registers, to the more-severe effects such as latchup or burnout that canIn the case of single-event risk, space electronics designers do their best either to acquire components that are radiation-hardened by design or that are shielded from charged particles, or design subsystems with error-correction approaches.
The same idea applies to risks from single-event latchup or burnout, which typically can happen when vulnerable electronics devices are subject to high radiation levels for long durations, which the industry refers to as total-dose radiation.
"In the case of flight-critical components, we try to increase radiation requirements to the highest levels possible in a manned-space mission," VPT's Leslie says.
The humans involved, and their vulnerability to radiation, are the primary reasons that human-crewed space missions will require much higher levels of radiation resistance than do unmanned satellites and deep-space probes. Space authorities want to avoid risks to human life in space as their highest goal.
"Unmanned missions may not be as risk-averse, like a satellite that is not high-value, where single-event performance can be reduced," VPT's Leslie says. "Even short-duration manned missions generally increase the single-event performance requirements to the highest levels for safety."
Priority on crew safety
When it comes to the safety of human space crews, "radiation-hardened is the biggest challenge of space flight," Leslie continues. "You get into varied radiation environments even within orbit. In low-Earth orbit you are under the radiation belts for the most part. But once you get beyond low-Earth orbit, you start to get into higher radiation levels from the Van Allen belt. Once you are outside of that, the radiation levels will fall off, but always pose the risk of single-event effects from the sun or other sources in the galaxy. It depends on location and duration of the mission."
While U.S. and international space authorities are planning manned space missions to the moon and later to Mars, some places in Earth's galaxy may pose radiation challenges that as yet cannot be met.
"One location that is difficult for manned mission -- almost impossible -- would be close to Jupiter, where the radiation levels are very high," VPT's Leslie points out. "We must do significant radiation hardening for even the unmanned probes that go toward Jupiter, because of the magnetic fields there. Jupiter has a significantly stronger magnetic field than Earth does."
The enabling technologies in electronics radiation hardening necessary for such a manned near-Jupiter mission are yet to be developed -- and perhaps never be sufficient for manned Jupiter missions -- at least not without substantial shielding and other protective equipment.
"You would need to have electronics that could stand that environment if you were to spend any time near Jupiter," VPT's Leslie says. "There would be significant challenges to have manned missions in that vicinity. Poorly performing radiation-hardened parts could stand a kilorad of total-dose radiation, but that level is lethal to a human."
One approach to mitigate radiation exposure near Jupiter might involve highly elliptical orbits to give humans and electronics a periodic break from Jupiter's intense radiation.
“For manned space flights, the level of reliability based on the margin you put into electronics is greater than for non-manned space flights," says Pratish Shah, general manager of Aitech North America, an embedded computing and radiation-hardened electronics specialist in Chatsworth, Calif.
Shah points out that unmanned spacecraft electronics can operate well at temperatures of 80 or 90 degrees Celsius (176 to 194 degrees Fahrenheit). Manned missions would require more stringent requirements. "Mankind can't survive at 80 degrees C," he says.
Transport and habitat
Manned space experts like Shah divide manned spaceflight into two basic applications -- both of which require life- and mission-critical radiation-hardened electronics: transport and habitat. Transport involves propulsion, navigation and guidance, sensors, and other systems to get humans safely into space and back"The transportation aspect is how you get someone from here on the ground to low-Earth orbit or to lunar orbit, says Aitech's Shah. "For the transport of individuals, the requirements are very stringent. The amount of pace you have in the capsule and the rocket, and the information that needs to be conveyed and acted on. The shock and vibration, environmental aspects, and acceleration impose stringent requirements in a very big way."
Habitat involves creating a human-friendly environment in space that will help enable humans to establish a more permanent presence in space.
"Everything that is life-critical requires redundancy and radiation hardness for extended-life operation," Aitech's Shah says. "Anything that controls life-critical systems will be subject to the most stringent requirements."
Where spacecraft designers might be able to compromise on radiation-hardening requirements might involve space experiments and other space activities that might not put human lives in jeopardy in the event of system failures or malfunctions. Using only radiation-tolerant electronic technologies in these areas has the potential to save money.
The lessons of NewSpace
In many ways, the legacy of recent technological developments in the commercialization of space are beginning to present tangible benefits to future manned space missions. Experiments in small orbiting satellites for telecommunications, cable television, and other commercial uses are teaching space systems designers about where they can compromise on stringent electronics requirements, and where they can't.
So-called "NewSpace" refers to the emergence of the private spaceflight industry, spanning areas such as private launch companies, small satellite constellations,Recent experiences of placing pure-COTS parts in space has led NewSpace designers to put more thought into some levels of ruggedization for space components. This is not to say the COTS approach has been abandoned -- far from it -- but industry consensus is coming around to some levels of value-add for space parts.
Systems designers have found that using pure-COTS parts can be risky, depending on the application, and has led to unanticipated on-orbit failures that can destroy or degrade the performance of NewSpace satellites. The solution often involves starting with pure-COTS parts and designing a test and measurement regimen to help prevent unexpected on-orbit systems failures or performance degradation.
Some electronics companies are turning to automotive-qualified electronic components from different chip fabs, can provide adequate ruggedization to survive the space environment. The trick is testing just enough to meet the application and the radiation environment.
Negotiating the middle ground between expensive and time-consuming radiation testing and non-tested full-COTS use can provide fast time to market and can less expensive than extensive testing. Selective radiation testing also encourages space systems designers to pay close attention to specific space applications that may or may not require addition radiation testing.
For unmanned commercial space endeavors, systems designers are finding ways to balance the risks, costs, and potential payoffs of which parts they choose ultimately for their spacecraft.
Lessons also are coming from the different orbits in which NewSpace satellites must operate, ranging from relatively benign low-Earth orbit (LEO) to geosynchronous and polar orbits, which have much higher exposure to space radiation.
In short, NewSpace is teaching spacecraft designers about how commercial and ruggedized electronics perform in space, where they could be used safely for non-life-critical applications, and where they cannot. NewSpace trends in determining just the right amount of testing and shielding for different space environments have the potential for bit payoffs in future manned space missions.
"Without a doubt, we are seeing that trend today for space transport and habitat in the transition to COTS for manned space," says Aitech's Shah. "We can benefit from the experiences of commercial and NewSpace. Unmanned flights have hundreds and thousands of hours of real-life experience in space. This is very important -- and perhaps is more important that analysis in the theoretical environment."
Putting plans into action
One of the most significant steps toward the future of human space missions happened in late 2022 with the Artemis 1 mission, launched by the U.S. National Aeronautics and Space Administration (NASA) from the Kennedy Space Center at Cape Canaveral, Fla. The goal of the 25-day mission was to test the Orion spacecraft and its heat shield in preparation for future Artemis missions to reestablish a human presence on the moon and demonstrate enabling technologies for future scientific studies, which may include a manned mission to Mars.
The Orion spacecraft then returned and reentered the Earth's atmosphere with the protection of its heat shield, and splashed down in the Pacific Ocean on 11 Dec.
Artemis I was the first in a series of increasingly complex missions to provide a foundation for human deep-space exploration. The mission traveled 280,000 miles from Earth and 40,000 miles beyond the far side of the moon.
With Artemis I, NASA sets the stage for human exploration into deep space, where astronauts will build and begin testing the systems near the moon needed for lunar surface missions and exploration to other destinations farther from Earth, including Mars.
For Artemis I, NASA’s Near Space Network and NASA’s Deep Space Network supported communications and navigation to enable flight controllers to send commands to the spacecraft and receive data from the spacecraft. Navigation enables the flight controllers to calculate the location of the spacecraft along its way through space.
The mission Near Space Network’s Launch Communications Segment provided links to Orion and its launcher on the ground, and NASA's constellation of Tracking and Data Relay Satellites (TDRS) provided near-continuous communications during launch and low-Earth orbit phases. Then NASA’s Deep Space Network took over to provide communications during Orion’s return to Earth, final return trajectory, and splashdown.
Lead contractors for the Artemis 1 rocket, spacecraft, and ground-support equipment are the Lockheed Martin Corp. Space segment in Denver; Aerojet Rocketdyne in Sacramento, Calif.; Northrop Grumman Corp. in Falls Church, Va.; The Boeing Co. in Chicago; Teledyne Brown Engineering in Huntsville, Ala.; and Jacobs in Dallas.
The European Service Module is the European Space Agency (ESA) contribution to NASA’s Orion spacecraft. It provides electricity, water, oxygen, nitrogen, andThe Orion crew module comes from Lockheed Martin, and integrates the European Service Module into a completed Orion spacecraft. Lockheed Martin performs the majority of the Orion engineering work in Denver, and manufactures the crew module pressure vessel and thermal protection materials, and assembles the spacecraft at Kennedy Space Center, Fla.
Aerojet Rocketdyne is the lead contractor for the four RS-25 engines that propelled the Artemis 1 mission during its 8.5-minute climb to space, and the RL10 engine that powers the rocket’s interim cryogenic propulsion stage.
Boeing is the lead contractor for the rocket's core stage and flight avionics. Northrop Grumman designs the twin solid rocket boosters that provide nearly 80 percent of initial thrust. Teledyne Brown Engineering designs the launch vehicle stage adapter, providing engineering, technical support, and hardware for the Artemis I adapter and structural test article. Jacobs builds the NASA Exploration Ground Systems Program.
Several follow-on missions are on the schedule. Artemis 2 will perform a crewed lunar flyby in 2024, and then Artemis 3, set for launch in 2025, will be the second crewed Artemis mission and the first crewed lunar landing since Apollo 17 in 1972.
Upcoming manned space launches
There will be three NASA-related manned space missions next year. The NASA SpaceX Crew-6 launch is set for mid-February 2023 to launch a crew to the International Space Station.
In April 2023 the NASA Crew Flight Test will demonstrate the ability of Starliner and the United Launch Alliance’s Atlas V rocket to carry astronauts safely to and from the International Space Station. Following a successful test flight with astronauts, NASA will begin certifying the Starliner spacecraft and systems for regular crew rotation flights to the space station.
Five space missions related to manned space flight are on schedule to launch over the next two years. In March 2023 the Intuitive Machines Commercial Payload Lunar Services project will launch robotic NASA payloads to the lunar surface to help prove the feasibility of delivering commercial payloads to the moon.
In mid-2023 the Lunar Trailblazer satellite will launch to provide an understanding of the form, abundance, and distribution of water on the moon, as well as the lunar water cycle. Also in 2023 nine science and technology instruments will launch to the moon's south pole.
2023 also will see launch of the JUpiter ICy moons Explorer (JUICE) European Space Agency spacecraft to carry the most powerful scientific payload ever flown to study Jupiter and its moons to help scientists understand how habitable worlds might emerge around gas giant planets.
November 2024 will see launch of the Astrobotic Commercial Lunar Payload Services robotic NASA payloads to the lunar surface as part of a Commercial Lunar Payload Services delivery.
The importance of robotics
Manned space flight and robotics often are considered to be separate topics, but in reality the future of manned space will rely heavily on robotics to handle danger, dirty, and dull jobs in space, as well as to help pave the way for humans to explore and inhabit the moon and other planets in the solar system.
A 2018 report of the International Space Exploration Coordination Group (ISECG) in Montreal, an organization of 27 global government space authorities, points out the importance of robotics in the future of human space flight.
"A partnership between humans and robots is essential in the success of this venture [manned space exploration]," states the 2018 ISECG report, The Global Exploration Roadmap. "Robotic missions accomplish world-class science while also servicing as our scouts and proxies, venturing first into hostile environmentsISECG members are the AEB in Brazil; AEM in Mexico; ASA and CSIRO in Australia; ASI in Italy; CNES in France; CNSA in China; CSA in Canada; DLR in Germany; the European Space Agency in Paris; GISTDA in Thailand; ISRO in India; JAXA in Japan; KARI in the Republic of Korea; LSA in Luxembourg; NASA in the U.S.; NOSA in Norway; NZSA in New Zealand; POLSA in Poland; PT Space in Portugal; ROSA in Romania; Roscosmos in Russia; SSAU in Ukraine; SSO in Switzerland; UAE Space Agency in the United Arab Emirates; UK Space Agency in United Kingdom and VNSC in Vietnam.
"In this global vision, robotic missions precede human explorers to the moon, near-Earth asteroids, and Mars in order to unveil many of their secrets, characterize their environments, and identify risk and potential resources," the report states. "Human exploration then follows in a coordinated manner that is affordable and sustainable, which both benefits and contributes to space agencies around the world achieving their goals and objectives."
Robotics research is being conducted for space-based remote manipulation, medical care, plant research for space food supplies, and related missions. NASA and other international space agencies also are developing robotic prototypes of lunar and Mars landers to prove-out enabling technologies before placing humans on those planets.
Who's who in manned space electronics
3D Plus, a Heico company
San Leandro, Calif.
https://www.3d-plus.com/index.php
Aerojet Rocketdyne
Sacramento, Calif.
https://www.rocket.com
Aitech
Chatsworth, Calif.
http://www.rugged.com
Apogee Semiconductor
Plano, Texas
https://apogeesemi.com
BAE Systems
Manassas, Va.
https://www.baesystems.com/en-us/productfamily/space-systems
The Boeing Co.
Chicago
https://www.boeing.com/space/
Cicoil Corp.
Valencia, Calif.
https://www.cicoil.com
Cobham Advanced Electronic Solutions Inc. (CAES)
Colorado Springs, Colo.
https://caes.com
Curtiss-Wright Defense Solutions Aerospace Instrumentation
Newtown, Pa.
https://www.curtisswrightds.com/company/locations-newtown.html
Data Device Corp. (DDC)
Bohemia, N.Y.
http://www.ddc-web.com
Dayton T. Brown Inc.
Bohemia, N.Y.
https://www.dtb.com
GSI Technology Inc.
Sunnyvale, Calif.
https://www.gsitechnology.com
Honeywell Aerospace
Clearwater, Fla.
https://aerospace.honeywell.com/en/markets/space
Infineon Technologies
El Segundo, Calif.
https://www.infineon.com
Jacobs
Dallas
https://www.jacobs.com
LEMO USA Inc.
Rohnert Park, Calif.
https://www.lemo.com/en
Lockheed Martin Corp. Space segment
Denver
https://www.lockheedmartin.com/en-us/capabilities/space.html
Lucid Circuit Ind.
Santa Monica, Calif.
https://lucidcircuit.com
Maxwell Technologies
San Diego
http://www.maxwell.com
Mercury Systems
Phoenix
https://www.mrcy.com
Microchip Technology Inc.
Chandler, Ariz.
https://www.microchip.com
Microelectronics Research Development Corp.
Colorado Springs, Colo.
http://www.micro-rdc.com/index.htm
Micropac Industries Inc.
Garland, Texas
https://www.micropac.com/markets/space
Microsemi
Aliso Viejo, Calif
https://www.microsemi.com
Nissha GSI Technologies
Burr Ridge, Ill.
https://www.gsitech.com
Northrop Grumman Corp.
Manhattan Beach, Calif.
http://www.northropgrumman.com
pSemi Corp.
San Diego
http://www.psemi.com
Qorvo
Greensboro, N.C.
https://www.qorvo.com
Radiation Test Solutions Inc.
Colorado Springs, Colo.
https://www.radiationtestsolutions.com/home
Renesas Electronics Corp.
Milpitas, Calif.
https://www.renesas.com/us/en/
Scientic Inc.
Huntsville, Ala.
https://www.scientic.com
Space Micro
San Diego
http://www.spacemicro.com/index.html
Spirit Electronics
Phoenix
https://www.spiritelectronics.com
Teledyne Brown Engineering
Huntsville, Ala.
https://www.tbe.com/en-us
Times Microwave Systems, an Amphenol company
Wallingford, Conn.
https://timesmicrowave.com
Triad Semiconductor Inc.
Winston-Salem, N.C.
https://www.triadsemi.com
VORAGO Technologies Inc.
Austin, Texas
https://www.voragotech.com
VPT Components
Lawrence, Mass.
https://www.vptcomponents.com
VPT Inc.
Blacksburg, Va.
http://www.vptpower.com
VPT Rad
Chelmsford, Mass.
http://www.vptrad.com
Xilinx Inc.
San Jose, Calif.
https://www.xilinx.com
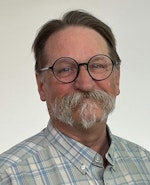
John Keller | Editor-in-Chief
John Keller is the Editor-in-Chief, Military & Aerospace Electronics Magazine--provides extensive coverage and analysis of enabling electronics and optoelectronic technologies in military, space and commercial aviation applications. John has been a member of the Military & Aerospace Electronics staff since 1989 and chief editor since 1995.